PATHOGENS AND BIOFUELS OFFER BIG QUESTIONS THAT MOTIVATE ERIKA TAYLOR
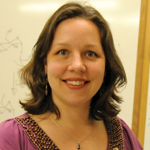
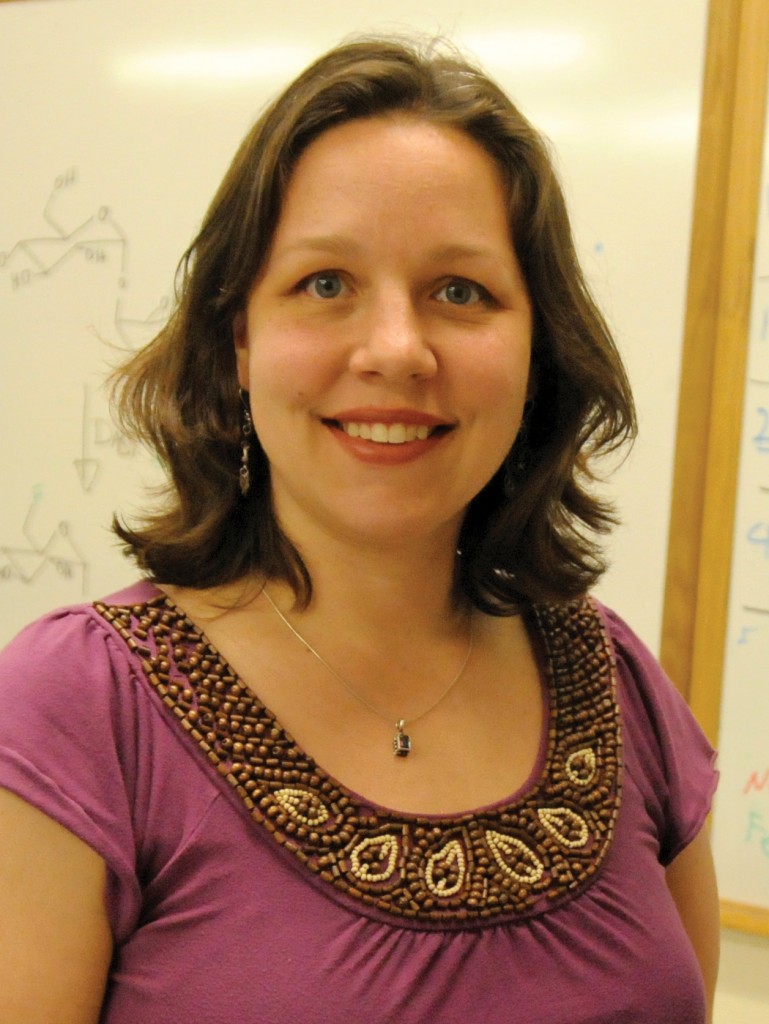
As only a fourth grader exploring in her backyard, Erika Taylor began her career as a scientist with an experiment. Now an assistant professor of chemistry and of environmental studies, she is digging deep into the inner workings of enzymes. Her goal is to uncover new ways to tackle foodborne pathogens like E. coli, and also to figure out how to make biofuels by using something other than corn.
As soon as I was starting to critically evaluate life, I began to think like a scientist. My father is a physician and my mother has a degree in biology, so we had a lot of conversations that included science.
The first time I set up an experiment was in fourth grade. We had a large garden and my job was to pick up all the rocks that were smaller than my fingers when I made a little circle. And so every couple of days I would go back out and there would be more rocks. I thought, These rocks must be growing. My mom said, “That’s interesting. What do you think makes them grow?” She wanted to let me show her how I was thinking about this observation.
So I got a bunch of Styrofoam cups and I put in rocks and the sand that they were coming from, so they were in the natural environment. I put one on the back porch and another in a part that had shade and one in the fridge, and here, there and everywhere. I remember thinking, Okay: these are all the variables I have to control for and these are all the things that could be different, that could impact this. I was thinking scientifically and that’s the first moment when I look back and see that I was headed toward science.
After two weeks I checked and the rocks were all still small. My mom said, “Let’s get an encyclopedia out and read about how rocks are formed and think about what is already known.” So the very first experiment I set up was a total failure, because I was missing key background information.
This early experience inspired me to help create the Girls in Science Camp that I run through a collaboration with Green Street Teaching and Learning Center and Professors Ruth Johnson and Christina Othon. I’m excited to be working with fourth- through sixth-grade girls because I know from my own experience how pivotal it was to have someone encouraging me to think about things scientifically at that age.
Each step of Taylor’s educational journey shaped her path toward the intersection of biology and chemistry and got her interested in work related to what she does today.
My organic chemistry class at the University of Michigan was the first class that excited me because I could see a lifetime’s worth of challenges and problems to overcome. After that class, I found a lab where I could do synthetic organic chemistry research.
I did synthetic research on sugar-based compounds and I learned that sugar synthesis is very, very hard. Sugars have a complicated three-dimensional structure, and small changes in experimental conditions can cause unintended changes in the stereochemistry or positioning of molecular groups.
Her experience as a graduate student shaped the philosophy she uses in her lab today.
My mentor’s philosophy was that you should study everything applicable to your project. I really liked that: It’s good to let your questions lead you to techniques.
As a postdoc, she moved into medical research at Albert Einstein College of Medicine.
While I had received very good training as a graduate student, the project wasn’t of global importance; it wasn’t a medical problem. I knew my professor could present me with problems that would be medically applicable and, if our solutions were successful, could have a very broad impact. That was what I thought I wanted.
My professor assigned me to his project on malaria. Five hundred million people are infected every year; in one year almost half a million children under five will die from the disease.
We hoped to gain better insights by examining all of the lifecycle stages of the disease. I studied enzymes from the malarial parasite, I studied enzymes from the mosquito, and I studied enzymes from humans. I even did some experiments with mice, to test the bioavailability of our inhibitor compounds; however, the point of the experiment was finding the dose that kills half the mice. I learned that I didn’t mind working with malarial parasites, but I didn’t want to work with mice; I couldn’t handle experiments whose intention was to kill something.
You have to figure out where your comfort zone is. Here in my lab I am trying to combine the “learn whatever you need to learn” philosophy with “let’s study problems with broad implications and let’s think about pathogens but not about mice.”
Taylor has continued to pursue research with medical implications at Wesleyan since 2007. In June she was awarded an NIH grant for $492,000 to continue her work on food- borne pathogens.
I have two big questions: The first is how can we make useful therapeutics for gram-negative bacteria, such as E. coli, Salmonella, and Vibrio cholerae. There are existing therapeutics that are used to treat gram-negative bacterial infections, but due to the bacteria evolving resistance mechanisms they are becoming less useful. My research involves designing inhibitor molecules for new targets in a biosynthetic pathway. Inhibition of these enzyme targets should lead to reduced pathogenicity of the bacteria, while also conferring renewed efficacy for existing antibiotics administered simultaneously. Also, our design strategy involves making inhibitors that look similar to bacterial metabolites, so as to trick the bacteria into taking them up.
A sub-question of this project goes back to my undergraduate research experience making sugar-containing compounds. I thought, Wouldn’t it be useful to make new enzymes that can add sugars onto something? I could modify my enzymes to make them tools for synthesizing sugar-containing compounds. Many sugar-containing compounds are important medically, functioning as drugs (like erythromycin, an antibiotic; or Bleomycin, a cancer chemotherapy agent) or other therapeutics (like heparin, an anticoagulant). Also, since all cells have sugars attached to their surfaces, being able to synthetically make sugar-containing compounds has a variety of uses, including production of substances that boost the body’s immune response to a vaccine.
Taylor’s work is unusual because she is also using the same tools to look for ways to make biofuel that avoid the typical route of turning corn into ethanol.
My second big question was inspired by seeing a flurry of announcements for biofuel funding initiatives—this was 2007. I through, I can come up with a biofuels project using my same tool set. Then I will have opportunities to look for funding from not only the NIH for my health-related project, but also from the Department of Energy.
The big problem today in biofuels is trying to efficiently convert whatever you are starting with to a biofuel. Having gone to graduate school in Illinois, I was aware of a lot of people who think about the conversion of corn into ethanol. The process is mostly about the kernels, the sugar in the kernels of the corn that are converted into biofuels, but most of the rest of the plant is a waste product. I wanted to focus on the other parts of corn, as well as other sources of biomass.
I picked the enzyme that we primarily study because the chemistry it does is hard. It’s not something that would be easy for me to do using synthetic organic chemistry approaches in the lab, so I thought it would be important to study how a biochemical system performs the reaction. I like the reaction: it is hard for a chemist to do, but easy for an enzyme to do.
Her efforts are focused on turning lignin, a polymer found in plants, into biofuel.
The tools we use are almost entirely the same as for the foodborne pathogen project, but the system is just very different.
What’s crazy about lignin is it uses three different building blocks and has more than 16 different ways of assembling these blocks, so it’s a crazy polymer. One molecule of this polymer is as big as the ring of a tree. It really stretches your imagination to think about the size of this molecule, since each new ring spans the length from roots to tip of a tree.
We’re interested in trying to understand the breakdown of lignin as a carbon source. We think the enzymes that degrade lignin could be mutated and engineered in ways that could have future industrial uses. Most of the work that is being done converting corn into ethanol uses the sugar in the corn as a carbon source, which gets turned into ethanol. Lignin has the advantage of not being a source of food, yet it’s abundant, making up 25 percent of all non-fossil fuel organic carbon on the planet. Lignin is found in trees and all other terrestrial plants. I liked it better than sugar as a starting material for these bacteria and yeast to make a fuel.
I feel like I have gone far enough into both of these projects to make an impact on the fields with some new discoveries and am excited about where my trajectory is taking me.