A Brain for Alzheimer’s
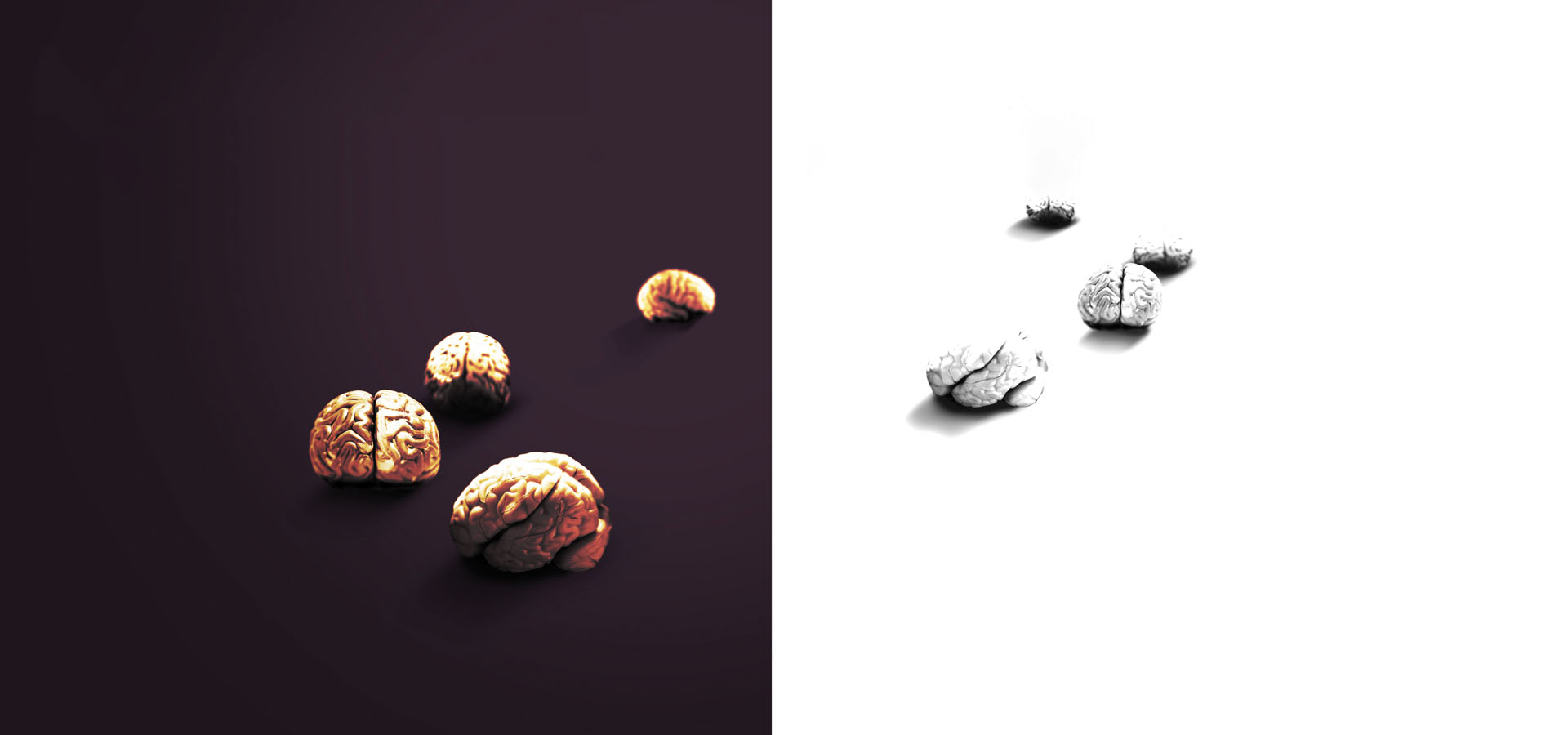
A biochemist with a self-described “neuroscience problem,” Professor Alison O’Neil is combating Alzheimer’s with a novel approach that bridges the sciences—and starts with a thousand tiny brains.
It’s lunchtime on a Thursday. Professor Alison O’Neil opens what resembles a wine fridge and removes something that’s been growing in there for the last week: a human brain.
Well, not a brain. The microscopic glob in O’Neil’s petri dish will never have blood vessels or immune cells. It’s not capable of thought or accidentally sparking a true-life sci-fi tale. But in a few months this miniature brain and about a thousand others growing in O’Neil’s lab will reach the size of peas, growing neurons that communicate with each other and organize into structures like a cerebral cortex, all on their own. “It’s a very simplified version of a human brain that has some of the important pieces,” says O’Neil, an assistant professor of chemistry at Wesleyan and an affiliated member of the Neuroscience and Behavior Program. “It’s like if a kindergartner made a brain.”
These mini-brains—properly called brain organoids—could be the key to understanding Alzheimer’s disease, a tragic condition that affects more than six million Americans. Using a special culturing technique and adult pluripotent stem cells derived from Alzheimer’s patients, O’Neil is searching for the moment when her brain organoids begin to develop the telltale, neuron-killing clumps of tau and beta-amyloid proteins believed to cause Alzheimer’s. It is a novel approach, one that could have major implications for the disease and has garnered O’Neil a five-year, $1.7 million grant from the National Institutes of Health (NIH).
By using these models to locate the elusive early stages that trigger patients’ descent toward dementia and death, O’Neil’s research could help lead to better treatments and prevention. “The goal of this research is to make a biochemical timeline where we can say, here is where Alzheimer’s ‘started,’ or where the pathology associated with mental decline [first] occurs—what was the step before that?” O’Neil says. “Because that’s where you need to treat it.”
An Unusual Perspective
In her office in the Hall-Atwater building, O’Neil recounts her academic journey from upstate New York to Montana State University, where she earned a PhD in protein biochemistry—the study of how proteins’ structure influences their function. But she went in a new direction for her postdoctoral work: in 2013, just as adult-induced pluripotent stem cells became a buzzy scientific frontier, O’Neil began working in cutting-edge labs at Harvard University and the Broad Institute. Instead of looking at proteins in the isolation of test tubes, she studied them within complex biological systems, cultivating brain organoids from stem cells in order to study neurodegenerative diseases like Parkinson’s and amyotrophic lateral sclerosis (ALS).
With an unconventional background compared to her cell biologist colleagues, O’Neil’s skills enabled her to see individual proteins through a different lens—a trait that she brought to Wesleyan upon joining the faculty in summer 2018. “Alison brings a cross-disciplinary way of thinking,” says Janice Naegele, dean of the natural sciences and mathematics and one of O’Neil’s mentors. “It’s that kind of thinking across borders that allows her to come up with fresh approaches to studying old problems.”
The O’Neil Lab initially focused on ALS, in which protein aggregations break connections between the brain and muscles by harming motor neurons in the spine. (This research hasn’t stopped: led by Wesleyan student Dan Kulick ’21, MA ’22, the O’Neil Lab recently published a paper in ACS Chemical Neuroscience showing that the pesticide cis-chlordane may cause ALS.) While growing clumps of motor neurons from ALS stem cells in a spinning bioreactor, O’Neil discovered the motor neurons eventually developed ALS’s hallmark protein aggregates—something that occurred in the oxygen-rich environment of the spinning bioreactor, but not in a petri dish.
Though she’d yet to study Alzheimer’s, O’Neil understood its parallels with ALS. She also had a hunch that growing cortical neurons—nerve cells that, unlike those clumps of motor neurons, can naturally organize into layers of the brain—inside a spinning bioreactor would create brain organoids with Alzheimer’s protein aggregates, potentially offering a way to glimpse the mysterious genesis of an insidious disease.
“We know what Alzheimer’s looks like after you get it, and we know what your brain looks like after you pass away because we have postmortem brain samples,” O’Neil says. “But for the most part, we really don’t understand the early stages.”
With the assistance of two PhD candidates and seven undergraduates, O’Neil’s study may change that while also providing opportunities for students to gain valuable, hands-on research experience with stem cells. “Looking at them and tracing them through the process of differentiation, where they become specialized cells, makes you feel how far we’ve come as scientists and how far this can take us in the future,” says Manaal Fatima, a second-year PhD student growing brain organoids as part of O’Neil’s Alzheimer’s research. “And hopefully our research can be something that actually makes an impact.”
Beyond Boundaries
The NIH’s Stephen I. Katz Early Stage Investigator Research Project Grant is an unusual source of funding, explicitly designed for scientists to take on projects outside their normal scope. But that’s precisely why it’s so valuable, O’Neil says: it encourages scientists to engage with “big-risk-big-reward kind of research” that expands beyond their field’s arbitrary boundaries. “We used to really silo science—‘you’re a chemist, you’re a biologist.’ But now the overlap is just growing,” she adds. “I’m in the chemistry department because I’m a biochemist and I’m interested in proteins, but I have a neuroscience problem.”
In early November 2022, after using grant funding to secure lines of stem cells containing the mutation that produces Alzheimer’s, O’Neil began growing brain organoids. Once their neurons begin to mature (about three months), her lab will look for clues into what makes beta-amyloid and tau proteins initially clump together and turn toxic—and when exactly that happens. For example, if a beta-amyloid plaque appears in a mini-brain after six weeks, O’Neil’s team can look back and investigate whether a polymer that surfaced two weeks earlier could have been a precursor.
“This is us bringing two silos together,” says O’Neil. “We have the biological tools and skills to make [organoids], but we also have a biochemistry lab to really understand the protein on a biochemical level.”
Today, O’Neil is outwardly excited about the ramifications of her research. Though there are questions around aducanumab—a new FDA-approved Alzheimer’s therapy that clears beta-amyloid plaques but hasn’t been definitively shown to improve cognition—O’Neil sees suggestions of potential; perhaps using it earlier in the disease’s advance could improve its efficacy, she says. Regardless, establishing a biochemical timeline is critical for a cure: The first signs of memory loss only appear after damage is done.
“Aside from COVID, Alzheimer’s is probably the biggest health problem right now,” O’Neil says. “But we don’t know what causes it. We don’t have any reliable therapies for it. We don’t really have anything to offer people other than hope.” With time, something new could emerge: a path toward the end of Alzheimer’s, starting with a search for the beginning.
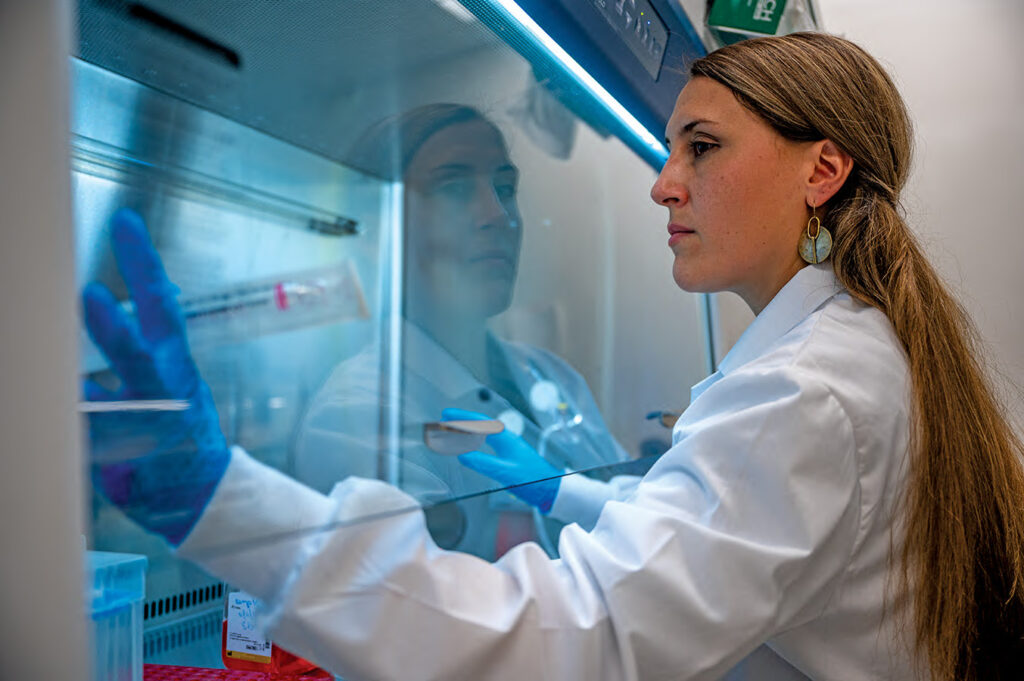
The Basics of Alzheimer’s
What happens in the brain: Toxic clumps of proteins—tau tangles and beta-amyloid plaques—accumulate and prevent nerve cells (cortical neurons) from functioning normally and communicating with one another. Usually starting in the brain region associated with memory (the hippocampus), the disease spreads, causing neurons to break down and die off while the brain itself literally shrinks.
What we see: Memory loss, personality changes, and other early signs of Alzheimer’s appear years or decades after irreparable cellular damage begins. As more parts of the brain are affected, patients suffer worsening cognitive and physical symptoms, including dementia and seizures. Eventually, this decline culminates in death.
What treatments are available: There is no known cure for Alzheimer’s. However, medications like galantamine and memantine can help patients manage symptoms. In 2021, after an accelerated review process, the FDA approved the Alzheimer’s drug aducanumab, a monoclonal antibody that clears beta-amyloid plaques; the controversial medication, however, has not conclusively been shown to improve clinical symptoms.
How to Grow a Mini-Brain
A miniature brain starts with an adult induced pluripotent stem cell—essentially, a full-grown cell that’s been coaxed back to its inception. By using a special combination of genes developed by Nobel Prize winner Shinya Yamanaka, skin cells return to pluripotency, after which they can take on new biological roles. In O’Neil’s case, cells donated from Alzheimer’s patients and acquired from stem cell banks become neurons.
Each brain organoid requires roughly 9,000 stem cells grown in suspension using a spinning bioreactor. Resembling a Nalgene bottle with a propeller, the device pulls in oxygen, delivers nutrients, moves waste away, prevents the cells from clumping together too much, and mimics the environment within a human body more closely than a petri dish.
After three to six months, neurons mature and self-assemble into brain structures as the brain organoid reaches the size of a green pea. (Though a brain organoid can theoretically grow indefinitely, it can’t grow any larger because it lacks blood vessels.) The fully realized mini-brain models have the same genetic background as the patients who donated skin, including the mutations leading to the protein aggregates associated with Alzheimer’s.